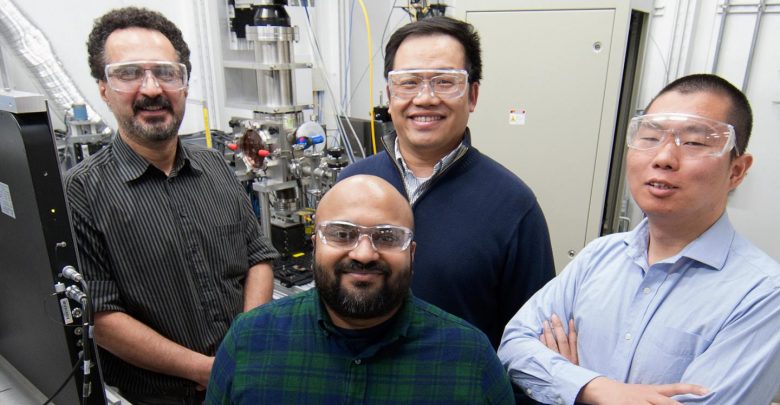
Researchers from Carnegie Mellon University and the Department of Energy’s Argonne National Laboratory have made a breakthrough in identifying how gas pockets form in parts during the additive manufacturing process. The research, which involved using high-energy X-rays to analyze the Laser Powder Bed Fusion (LPBF) process, could help eliminate the risk of defect-causing gas pockets from 3D printing.
One of the challenges currently plaguing metal 3D printing is the creation of defects in the print process, such as gas pockets, which can lead to cracks and performance compromises in the final part. The problem is presently standing in the way of additive manufacturing achieving true industrialization, especially for industries which have stringent part requirements.
Fortunately, a team of researchers from Carnegie Mellon and Argonne National Laboratory have made a breakthrough on this front, identifying how and when the harmful gas pockets form (known as the keyhole phenomenon). The team has also formulated a methodology which allows for the prediction of gas pocket formation, which could take powder-based metal AM to the next level.
“The research in this paper will translate into better quality and better control in working with the machines,” explained Anthony Rollett, a Professor of Materials Science and Engineering at Carnegie Mellon University and an author on the paper. “For additive manufacturing to really take off for the majority of companies, we need to improve the consistency of the finished products. This research is a major step in that direction.”
The breakthrough in addressing the keyhole phenomenon was largely thanks to specialized X-rays developed at Argonne. An “extremely bright, high-energy” X-ray system at the lab’s Advanced Photon Source (APS) enabled the researchers to capture rapid video and images of the LPBF process, which uses a laser to melt and fuse metal powder particles together.
This information provided greater insight into how gas pockets become trapped between fused layers of metal. Prior to this, the cause of these defects had been mostly attributed to the type of metal powder or strength of laser, which entailed a trial and error process on the part of manufacturers to minimize the defects.
It turns out, however, that the gas pockets (or vapor depressions) can form in nearly all print conditions. And though this may not sound incredibly hopeful, the insight has also provided researchers with the knowledge to predict how the depressions form and determine whether they will grow to become unstable and harmful to the part.
“We’re drawing back the veil and revealing what’s really going on,” Rollett said. “Most people think you shine a laser light on the surface of a metal powder, the light is absorbed by the material, and it melts the metal into a melt pool. In actuality, you’re really drilling a hole into the metal.”
The images of the LPBF process captured using the X-rays showed that the high-power laser does not always create a consistent melt pool shape. That is, while the perfect melt pool shape is shallow and semicircular, the laser can often change the shape to something resembling a keyhole in a warded lock. The researchers describe it further as “round and large on top, with a narrow spike at bottom.” It is these keyhole-shaped melt pools that can lead to gas pockets.
“Based on this research, we now know that the keyhole phenomenon is more important, in many ways, than the powder being used in additive manufacturing,” added Ross Cunningham, a recent graduate from Carnegie Mellon University and one of the co-first authors of this paper. “Our research shows that you can predict the factors that lead to a keyhole—which means you can also isolate those factors for better results.”
The factors in question arise when the laser power density increases to the point where it is strong enough to effectively boil the melted metal. This, the researchers explain, showcases the importance of laser focus in the AM process. They ultimately hope their discovery will encourage metal 3D printer manufacturers to integrate more flexibility in controlling their systems. More flexible control of features like the laser could result in fewer print flaws and even faster print times.
“We are really studying the most basic science problem, which is what happens to metal when you heat it up with a high-power laser,” said Cang Zhao, an Argonne postdoc and the other co-first author of the paper. “At the same time, because of our unique experimental capability, we are able to work with our collaborators on experiments that are really valuable to manufacturers.”
The research study, “Keyhole threshold and morphology in laser melting revealed by ultrahigh-speed x-ray imaging,” was recently published in the journal Science.