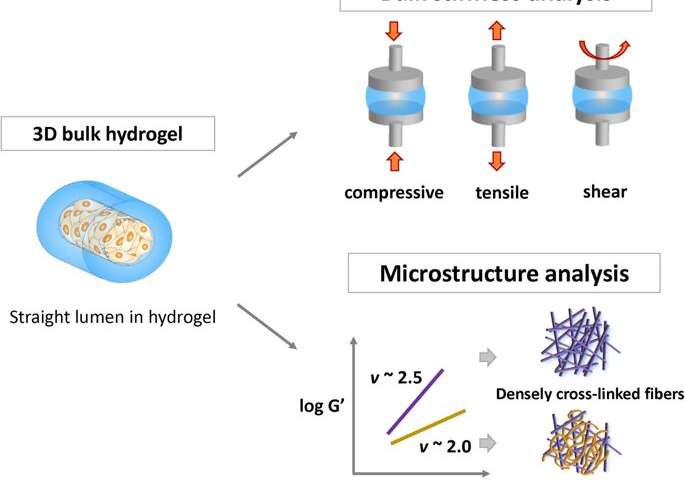
Hydrogels are commonly used as biomaterials for applications in biomedicine due to their biocompatibility. However, the relationship between biological cells and the hydrogel surface is still unclear and the existing parameters to explain the interactions are not sophisticated enough. In a recent study, Jooyoung Lee, Boa Song and co-workers at the Center for Biomaterials and the Department of Polymer Engineering in the Republic of Korea studied the impact of polymer chain flexibility on cell adhesion, with a variety of hydrogel constructs composed of the natural polymers collagen and fibrin.
They introduced a new method of semi-flexible, model-based analysis to confirm that chain flexibility mediated the hydrogel microstructure as a critical factor that allowed cell adhesion at the cell-material interface. The analysis proposed in the study is able to more accurately predict biocompatibility (cytocompatibility) of hydrogels. Results of the work now published in Scientific Reports, provide an important criterion for polymer design and development by enhancing biocompatibility and biofunctionalization at the cell-material interface for biomedical applications in vivo.
Hydrogels are made of polymer networks swollen with water and have applications in drug delivery and tissue engineering. Cell-material adhesion is crucial for in vivo biocompatibility and most studies have tested cell behavior by analyzing bulk stiffness of the materials composition. Nevertheless, communication between cells at the hydrogel surface remains to be accurately understood. The FDA approved natural polymers collagen and fibrin, provide excellent biocompatibility for biomedical applications. Also known as semi-flexible polymers, they do not comply with models of flexible chain solutions or rigid-rod networks. The semi-flexible model allows the prediction of chain flexibility of polymer networks by experimentally scaling the elastic plateau modulus.

In the new study, Lee and Song et al. proposed a new, semi-flexible model-based analysis to understand cell adhesion to hydrogels using the well characterized collagen and fibrin polymers. They used three different collagen and fibrin constituents, to investigate the factors that determined cell adhesion:
- Two dimensional (2-D) coated substrates.
- 2-D bulk hydrogels, and
- 3-D bulk hydrogels
The scientists varied the hydrogel concentration in the material constituents from 1 mg/mL to 7 mg/mL and quantified the bulk stiffness and roughness of the newly formed biomaterials. To define the parameters of cell attachment, they observed the chain morphology of the new materials. The results confirmed that the microarchitecture of hydrogels affected chain flexibility as a crucial factor affecting cell adhesion.

In the experimental setup, the scientists prepared a variety of collagen and fibrin constructs, to test cell adhesion without gravity effects. They controlled hydrogel stiffness using incremental concentrations of each component and quantified the stress-strain relationship based on axial (tensile or compressive) and rotational (shear) stress. To measure the rheological properties of the hydrogels, the scientists used a stress controlled rheometer. They then measured the compressive modulus for tensile testing with a universal testing machine. To investigate surface topography, the scientists used an atomic force microscope, followed by microindentation to measure the Young’s modulus (E) of the hydrogels, where they calculated average (E) using JPK data processing software. Lee and Song et al. believe the study was a first to investigate cell adhesion onto hydrogels using the slope of elasticity of model semi-flexible polymers.
For cell culture in the lab, Lee and Song et al. used human umbilical vein endothelial cells (HUVECs) as the preferred cell line. They measured the rate of cell adhesion on diverse concentrations of collagen and fibrin hydrogels in 2-D, and quantified cell adhesion using the cell counting kit 8 (CCK-8). The scientists did not observe a statistically significant difference between cell adhesion on 2-D collagen and fibrin coated substrates; possibly since cells sensed the mechanical properties of cell culture plates, instead of material properties of the hydrogels. In 2-D bulk hydrogel surfaces, cell adhesion to collagen (2-D bulk gels) was much higher than that on 2-D fibrin bulk gels. Furthermore, as the hydrogel concentration varied, the scientists observed the cell attachment systematically increased with the increasing concentration of collagen. In comparison, cell adhesion on fibrin gels was independent of the concentration of the incorporated hydrogel.

Lee and Song et al. then used 3-D lumen structures of hydrogels to clarify the rate of cell adhesion on collagen and fibrin gels. They observed the cells were better attached to collagen compared to fibrin gels. Cell adhesion phenotypes were also much clearer on the 3-D constructs compared to attachments on 2-D. Typically, cells attach to material surfaces or the extracellular matrix (ECM) via anchorage points known as focal adhesions. Cells on stiffer substrates generally contain well organized cytoskeletons for stable focal adhesions. To understand if bulk hydrogels could contribute to cell adhesion, the scientists plotted the bulk stiffness of the hydrogels and compared collagen and fibrin at different concentrations. Lee and Song et al. thereby experimentally showed that bulk stiffness was not a critical factor to impact cell adhesion onto the hydrogels.
The scientists determined the Young’s modulus and compressive modulus as additional mechanical properties of the materials that influenced cell adhesion. They showed how the stiffness increased with increasing concentration, and suggested chain flexibility as an appropriate parameter. Despite the increased stiffness with increasing concentration of collagen and fibrin, the scientists could not explain the varying cell adhesion rates observed between the two polymers.
For this, Lee and Song et al. examined the architecture of fiber molecules and their networks on the microscopic scale using atomic force microscopy. Collagen gels displayed a fibrous structure as reported before, with well-defined rod-shaped, semi-flexible filaments. In comparison, in the fibrin gel structure, the scientists only observed a few semi-flexible filaments, where the associated protein was entangled as dynamically trapped flexible chains. To confirm the degree of cell on hydrogel attachment, the scientists stained the cells with the cell surface marker CD31 (glycoprotein expressed on endothelial cells) and confirmed the stable architecture of the endothelium on the material.
The scientists observed that total cell attachment to collagen hydrogel was greater than on fibrin hydrogel. The outcomes were due to the higher stiffness of collagen at the microscopic scale, compared to the relatively flexible chain architecture of fibrin. The scientists thus showed the microscopic stiffness of hydrogels as a dominant factor that determined the degree of cell attachment onto a biomaterial surface.
In this way, Lee and Song et al. proposed analytical methods in the study to describe the interaction between cell adhesion and material surfaces. The results will provide significant guidelines during biomaterial design in the future, while integrating optimized material properties of durability and mechanical strength for hydrogel applications in vivo. The scientists demonstrated a semi-flexible, model-based explanation of cell adhesion to biomaterials by studying tissue stiffness to control cell adhesion, proliferation and differentiation on the material constructs. The authors propose that this simple method can explain the properties of cell adhesion on polymeric biomaterials for precise predictions of biocompatibility. The results will provide a practical tool to design and construct 3-D artificial tissue with higher biomechanical precision and biocompatibility for a variety of applications, such as bioengineering blood vessels and drug delivery mechanisms in vivo.