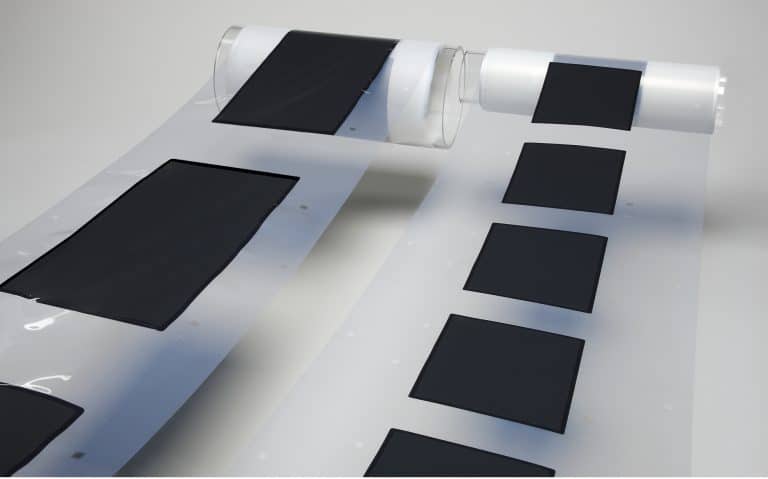
As the world moves toward reducing its dependency on fossil fuels, renewable energy generation sources such as wind and solar will be expected to fill the gap. Due to their cyclical nature, grid scale energy storage is essential for maximizing the utilization of renewable generation assets. It is not always possible to match peak generation times with peak usage, because they can vary on daily, monthly and seasonal scales. In addition to supplementing power distribution when demand is high, the energy storage technology must also store the over-generation of power for later use.
Of the myriad storage technologies available to fill this role, fuel cells and lithium-ion batteries stand out as promising candidates. While different in architecture, years of product research has spurred advances for both technologies.
Lithium-Ion Batteries
While often just described as a “lithium-ion” battery, the name represents a wide array of anode and cathode materials that are combined to allow for lithium-ion transport.
The first commercial example is the combination of a graphite anode with a lithium cobalt oxide (LCO) cathode that was used to power portable electronics. Since then the industry has seen LCO replaced by lithium nickel manganese cobalt oxide (NMC) and portions of graphite replaced by silicon to achieve improved safety and energy density.
Lithium-ion battery use in grid-scale applications has seen expansive growth over the past decade. Strong adoption has been observed in applications that require less than four hours of continuous operation and high-charge acceptance rates.
The rapid decline in system costs due to manufacturing advancements in the portable electronics industry has also contributed to adoption speed.
Going forward, researchers will continue to seek energy density, life, and safety improvements by drawing on 3M’s material science capabilities. Stretching the chemistry to higher voltages, improving manufacturing yields and better system understanding will continue to lead the industry to a very bright future.
Fuel cells
Fuel cells are electrochemical devices that convert chemical energy into electrical energy. Instead of using chemical reactants sealed in a battery-like container, fuel cells are open systems where gases are continually fed into the stack and the reaction byproducts removed. This is important because electricity generation can continue indefinitely if fresh reactants are supplied.
In contrast to gas engines, fuel cells primarily release relatively harmless byproducts like water and carbon dioxide into the atmosphere. For example, fuel cells that use hydrogen as the reductant and oxygen from the air as the oxidant generate electricity with water as the only by-product.
Fuel cell options are classified by the electrolyte used in the cell. Proton exchange membrane fuel cells operate at lower temperatures, less than 100°C. The electrolyte in this case is a polymer-based proton exchange membrane. These systems are suitable for backup power or transportation due to their ability to quickly respond to load demands.
As a storage device, fuel cells make use of excess generated electricity and water to form hydrogen gas—the opposite of the fuel cell reaction. The stored hydrogen can be used immediately for fuel cell vehicles or later for grid use.
Due to the large number of factors that need to be considered, choosing the proper energy storage system can be daunting. Run time, system footprint, initial and total lifetime operating costs are just a few factors that must be considered.
Where in some cases lithium-ion might be the optimum choice to address a daily deficiency, fuel cells could be a better option to meet a multi-day outage.
Most importantly, one must remember that there is no one perfect energy storage system. It will require a truly diverse set of technology solutions to meet the goal of a 100% renewable power grid.