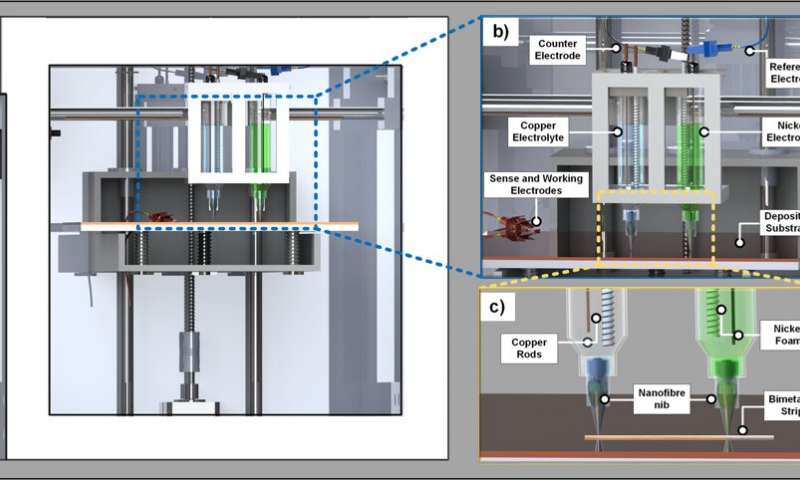
Four-dimensional (4-D) printing can create complex 3-D geometries that react to environmental stimuli, opening new design opportunities in materials science. A vast majority of 4-D printing approaches use polymer materials, which limit the operational temperature during the process of engineering. In a recent study, Xiaolong Chen and co-workers at the Dyson School of Design and Engineering, Department of Earth Science and Engineering and Department of Materials at the Imperial College of London, U.K., developed a new multi-metal electrochemical 3-D printer. The device was able to construct bimetallic geometries by selectively depositing different metals with temperature-responsive behavior programmed into the printed structure. In the study, they demonstrated a meniscus confined electrochemical 3-D printing approach using a multi-print head design and nickel and copper materials as examples, the ability can be transferred to other deposition solutions. The results are now published in Scientific Reports.
Additive manufacturing (AM), popularly known as 3-D printing, can fabricate complex 3-D architectures by sequentially joining materials layer-by-layer. The flexibility of AM has now found applications in the aerospace, automotive, medical and energy industries. At first, AM applications focused on the use of polymers to ease consolidation, either via photopolymerization (stereolithography) or thermal processes (such as fused deposition modeling: FDM). However, the increased uptake of metal-based AM has now transitioned the technology from a prototyping tool to engineering end products. The main technologies used for practical applications include:
- Direct-laser sintering
- Electron-beam melting
- Decreased energy deposition, and
- FDM using a metal filled polymer filament.
A majority of these methods only used a single material, while ongoing research efforts aim to expand methods to include multi-material capabilities. A main drawback of the previous methods for metal-based multi-materials engineering was the high capital cost of the experiment and the associated safety-risks with the use of high power lasers, metal powders and high temperature heat treatment.
Electrochemical additive manufacture (ECAM) is a relatively new technique of metal AM that can integrate localized electrochemical deposition of metal ions from electrolyte solutions to create metal structures. ECAM is advantageous as it eliminates thermal processes to offer a safer system at a lower cost, although challenges surrounding the deposition speed remain to be overcome. A novel design possibility included 4-D printing to create self-assembling and self-regulating structures that could change shape due to environmental stimuli such as temperature, humidity or light. 4-D structures are commonly fabricated by synthesizing active materials with temperature-responsive properties to control the thermal boundary conditions and achieve temporary shapes.
Existing multi-metal 3-D printing approaches are thermally based, where a blown powder or wire is fed into a melt pool created by a laser in an inert environment. Since metals have higher melting temperatures compared to polymers, it is possible to create 4-D structures with higher operating temperatures and mechanical strength with metal. However, researchers are yet to develop a low-cost multi-material metal printer. In the present work, Chen et al. presented a new ECAM-based approach to create multi-metal structures with high resolution and low cost. As examples of the merits of the approach, the scientists demonstrated programmed, mechanical responses to thermal stimuli by constructing copper-nickel bimetallic strips.
The new work followed previous experiments conducted by the same research team. In the present study they reported characterization techniques of the printed copper-nickel bimetallic strips, including electrical conductivity and surface morphology using standard techniques of scanning electron microscopy (SEM) and X-ray computed tomography (XCT). The scientists converted a commercial FDM 3-D printer into a low cost electrochemical multi-metal 3-D printer. The setup contained two syringes; each with copper sulphate electrolyte (blue) and the nickel sulphate electrolyte (green). They then inserted two copper wires into the copper sulphate electrolyte syringe assembly; one as a counter electrode and another as a reference. The nickel solution syringe was similar in composition, with nickel foams in place of copper wires. The movements of the setup were computer controlled.
During fabrication, Chen et al. filled one syringe with electrolyte for deposition, while the other remained empty to prevent undesirable mixing of the electrolyte solutions. In the first stage, they deposited a copper layer from the aqueous copper sulphate electrolyte to form a stable electrolyte meniscus between the nozzle and the substrate. The scientists then used a potentiostat to apply a constant potential and reduce Cu2+ ions in the electrolyte to metallic copper on the substrate. In the present work, Chen et al. used an electrospun nanofiber nib to faciliate the process. After depositing the copper layer, the scientists similarly deposited a nickel layer and obtained SEM images of the materials.
The scientists observed a clear interface between the nickel and copper surfaces, where both metallic layers showed polycrystalline or nanocrystalline morphology. The surfaces also contained a convex shape due to higher reaction current density during nozzle-based deposition. To investigate thermo-mechanical properties of the printed bimetallic strips, they placed the samples on a heated bed with one component fixed and the other free to move. Chen et al. then increased the temperature from 500C to 3000C and placed a camera above the samples to view the degree of displacement. Due to the diverse thermal expansion coefficients of copper and nickel, the scientists observed mechanical deformation of the materials, generating internal stresses in the metallic layers tightly bound at the interface. To detect the angle of deformation, they fit the acquired images to a circle and deduced the radius of curvature using MATLAB software.
Thermo-mechanical response of different copper (3 h, 5 V vs Cu)-nickel Ni (5 hr, 2 V vs Ni) structures fabricated through a multi-nozzle ECAM approach. (a) Deformation of a Cu-Ni bimetallic strip with perpendicular heating. (b) Deformation of a Cu-Ni bimetallic strip with selective nickel deposition in the center of the strip and heating with the strip flat against the heating bed. (c) Deformation of a Cu-Ni-Cu tri-layer strip with Cu-Ni-Cu sandwich structures at both ends of the strip. (d) Optical images of samples programmed to exhibit the letters “ICL” at room temperature and 300 °C “ICL”. The scale bar is the same to all images in length of 2 mm. Credit: Scientific Reports, doi: https://doi.org/10.1038/s41598-019-40774-5
Key design variables that affected the radius of curvature of the bimetallic strips included the layer thickness, Young’s modulus and the thermal expansion coefficient of the two layers as derived in the study. The scientists measured the bending angles for different Cu-Ni bimetallic strip compositions at different temperatures and characterized the samples with XCT reconstructions, SEM micrographs and EDS mapping at the material interface. Chen et al. measured the electrical conductivity of the bimetallic strips and implemented a simple electrical circuit actuated by the printed bimetallic strip. The printed bimetallic samples could function in high temperature environments as observed with the simple circuit. When the scientists increased the temperature to 3000C, the Cu-Ni bimetallic strip bent, closing power to the LED and showing its ability to sense the environment, opening new possibilities for smarter 3-D printed structures
In this way, Chen et al. developed a new electrochemical 3-D printer to engineer multi-metal (copper and nickel) temperature-responsive, 4-D structures. They characterized the tightly bound interface of Cu-Ni and programmed the angle of binding of the materials upon exposure to temperature. As a proof-of-concept, they constructed a simple temperature sensing circuit and designed thermal stimuli based structures of interest. The findings demonstrated the first reported, low cost, multi-metal 3-D printing approach to create high temperature 4-D structures. The research will open new possibilities to create intelligent and complex, 4-D self-assembling/actuating metal architectures and sensors at high temperatures using inexpensive components and multiple materials.